Animals and plants can use the pigment melanin to gain heat from their environment, maintaining comfortable body temperatures. This is known as thermal melanism. But melanin also serves other functions in biology. In humans, for example, melanin helps prevent exposure to ultraviolet radiation, so humans who live closer to the equator often have higher levels of melanin in their skin. When researchers at Johns Hopkins University Bloomberg School of Public Health wanted to study pigmentation in microbes, they hypothesized that the distribution of pigmentation in the world would be consistent with ultraviolet protection. Instead, a bioinformatic analysis of 693 yeast isolates indicated a geographical distribution consistent with the thermal melanism theory.
A team of researchers, led by Arturo Casadevall, studies the thermal tolerance of fungi, focusing on one particular species, Cryptococcus neoformans, which can live all over the globe and produce different colors. Using this model, they wanted to prove that microbial pigmentation can function in temperature regulation. The team discovered that yeasts with darker pigments absorb more heat and survive better in cold environments. “We’re extending a phenomenon that has only ever been documented in higher level metazoans to microorganisms,” explained Radames J.B. Cordero, lead author of the study.
In order to prove that microbes can use pigments for thermoregulation, they set out to answer three questions. First, having darker pigments should mean that the microbes will become hotter following exposure to light. Second, that this feature should affect fitness depending on ambient temperature. And finally, if pigmentation affects fitness, the team should find a geographic distribution of fungi depending on their color.
To test the first question, the team grew yeast with different pigments and exposed equal masses to different frequencies of light, including a white LED lamp, infrared, ultraviolet, and sunlight. At different time points, they took a thermal image that captures the intensity of the infrared photons being emitted by the fungus (blackbody radiation). They found that darkly pigmented fungi emitted more blackbody radiation, meaning these got hotter than lighter clones.
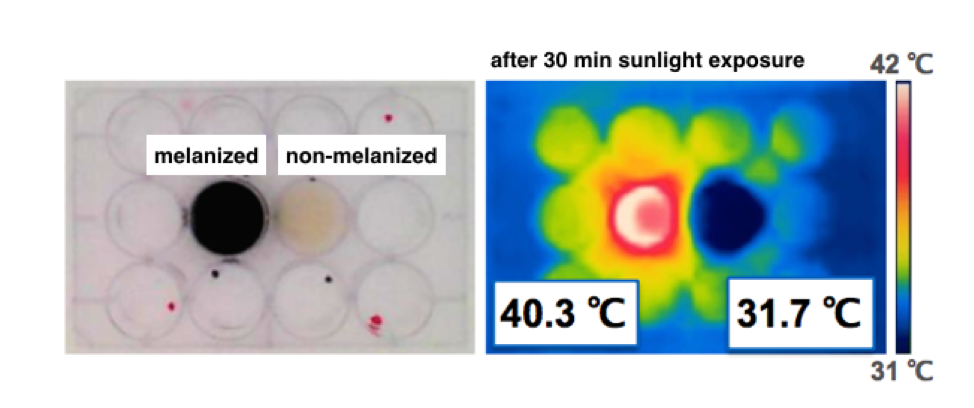
Melanized yeasts become hotter than non-melanized clones following exposure to sunlight.
Next, they measured out equal masses of melanized and non-melanized microbes and exposed them to white light under two different temperature settings. The fungus high in melanin got too hot and perished in the warmer environment but survived at higher rates in the colder environment. The inverse was observed for the non-melanized clones, as predicted.
Finally, the team used data from a global database of fungi to see how pigmented fungi were distributed across the globe. They found a statistically significant distribution of darker-pigmented fungi at higher latitudes. Consistent with the phenomenon of thermal melanism, microbes with darker pigmentation are frequently adapted to colder climates because they can absorb more heat.
Because pigmented microorganisms can thrive in cold areas, like on glaciers, microbes are another source for accelerated global warming. As temperatures warm due to climate change, microbes are more likely to thrive. These microbes absorb heat and warm up the ice, causing glaciers to melt. This can indirectly impact Chesapeake Bay because “the big concern of melting glaciers is sea level rise,” explained Cordero. “I think these studies are important to help estimate how much heat microbial communities are able to absorb and impact Earth’s energy balance.”
Next, Cordero wants to figure out what happens to the heat captured by melanin. Does melanin affect how the heat is dissipated? He predicts that this emerging research could lead to the birth of a new field: thermal micro-biology. Continuing research could help better understand the influence of pigmentation on how biological matter exchanges energy with its surroundings and better predict rates of glacial melting in the face of climate change.