When modeling low-oxygen environments, researchers examine chemical signals of biogeochemical cycles, but rarely do they try to model the microbial community underlying those cycles. Sarah Preheim of Johns Hopkins University (JHU) used a lake ecosystem to model how the microbial community responded to anoxic conditions. She hopes to one day apply techniques learned from the lake study to the entire Chesapeake Bay.
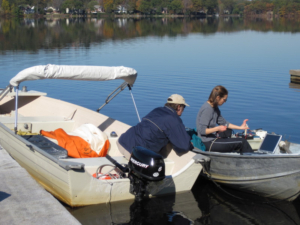
Preheim getting ready to start sampling on Mystic Lake. Credit: Sarah Preheim.
Preheim spent 6 months studying the Upper Mystic Lake in Medford, Massachusetts trying to understand how the microbial community responds to seasonally anoxic conditions. She and her team compared the observation of the microbial community to a biogeochemical model they built to see how well they could predict how the community responds to changing environments conditions.
She started this work when she was a post doc at MIT, looking at not only which microbes were present in the lake, but also their genetic makeup. At Upper Mystic Lake, researchers knew little about the composition of the microbial community, and even less about the combination of genes within genomes mediating biogeochemical processes. She wanted to take a quantitative approach to understand why organisms are found where they are and which processes they mediate at those locations.
One surprising thing her team found was that sulfur-cycling microorganisms are using nitrate to oxidize sulfide, connecting the sulfur and nitrogen cycles in a way that hasn’t been observed in the lake before. Organisms often oxidize sulfide to sulfate using oxygen, but when oxygen isn’t available, they turn to nitrate. This means these organisms are able to conduct complete denitrification, emitting nitrogen gas, which is not as bioavailable as nitrate, at the end of the cycle. This finding also means that sulfur-cycling microorganisms are competing with methane and iron oxidizers also using nitrate. This competition, which she demonstrates through the model, could have a substantial impact on the concentration of chemical species in the lake like oxidized iron, arsenic and phosphate.
Often when modeling anoxic conditions, researchers observe the chemistry and make inferences about what processes might be occurring. Now that they understand the community and its genetic makeup, “we have insight into how microbes are connecting these cycles in ways we couldn’t have known just from the chemistry,” Preheim explained. “From the chemistry, there’s no indication they’re connected.”
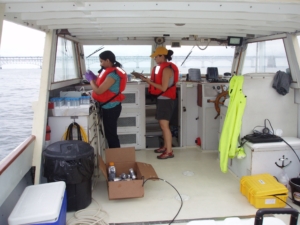
Preheim and students taking samples at Chesapeake Bay Bridge in 2015. Credit: Sarah Preheim
Sulfide oxidation is not known to be coupled to denitrification in the Chesapeake Bay, but preliminary data suggests this may be the case. Preheim hopes that she can use the observations from the microbial community and their genome composition to one day design a biogeochemical model that can be incorporated into the larger Chesapeake Bay model, including sulfide-oxidation coupled to denitrification. “It’s a career-long goal,” she explained. “It would need to be up to the standard of the amazing models that are already developed for the Bay.”
To meet that goal, she has already collected samples from the Bay with the Maryland Department of Natural Resources and Old Dominion University’s Water Quality Lab. She has sequenced hundreds of samples from the Bay between 2015 and 2017, which will provide data about the species composition, gene content and genome composition of microorganisms mediating biogeochemical cycles in the Bay. She is also hoping to start to apply a similar technique this summer on a low-oxygen site at Rock Creek, testing how microbes respond to periods of low and high oxygen, controlled with aeration.
Preheim explains that understanding the microbial community can provide important insight into other important processes in the Bay. “There are a lot of other things that microbial communities can tell us. Not only can they help explain chemical transformations within the dead zone, but potentially explain other water quality indicators, harmful algal blooms, and the distribution of antibiotic resistance genes in the Bay. Microbes can be an early indicator of something dramatic happening, maybe before you even see changes in the chemistry,” she explained. “Developing a quantitative understanding of factors that influence the microbial community composition and function will help us model their response to a multitude of environmental changes.”
You can read more of Preheim’s work in her paper:
Arora-Williams, K. et al. 2018. Dynamics of microbial populations mediating biogeochemical cycling in a freshwater lake. Microbiome 6: 165. https://doi.org/10.1186/s40168-018-0556-7