How Water Chemistry Changes in Response to Urbanization
Scientists have long known that land development can have significant negative impacts on local streams. Instead of focusing on water quality measured with a single grab sample, a team of researchers at University of Maryland Baltimore County (UMBC) looked at water chemistry over a 30-year time span as 12 watersheds were developed. Their results were more complicated than you’d think.
Why use conductivity to understand water quality?
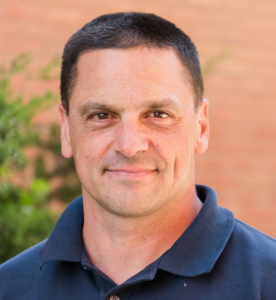
Matthew Baker. Photo courtesy of UMBC
Dr. Matthew Baker is a professor of geography and environmental systems interested in watershed science and factors that contribute to the degradation of stream ecosystems. This interest has led him to take a broader look at how water chemistry has changed in Maryland as impervious surfaces (e.g., roads, parking lots, rooftops) continue to expand around Baltimore-Washington, D.C.
He chose to use specific conductivity because, as a proxy for dissolved solids, it generally tracks water quality, and because the Maryland Department of Natural Resources has been measuring it in monthly water samples from a selected set of watersheds for the past 30-years. When pollutants enter waterways, most dissolve as conductive ions, raising the conductivity of the water.
“Conductivity has been used as an indicator of development because it’s relatively easy to measure and often associated with urbanization,” explained Baker. He added that while development can increase concentrations of nutrients like phosphorous and nitrogen, they are not the only materials added to waterways, noting that “increased conductivity is relatively ubiquitous downstream of all kinds of land development.”
Baker, former UMBC student Matt Schley, and colleague Joseph Sexton reviewed changes in impervious surface area across 12 watersheds in Maryland from 1985 to 2010. They used an annual impervious cover data set previously developed by Sexton and Baker from satellite imagery, then compared patterns of conductivity over the same time period. Not surprisingly, they found that conductivity increased during expansion of impervious surfaces. But they also found patterns of variability that led to a few interesting conclusions.
Conductivity and urbanization: an inconsistent relationship
In their paper, recently published in Water Resources Research, Baker and his co-authors show that as levels of watershed impervious surface increased from 0 upto 4-5%, conductivity increased sharply across all seasons with very limited variation across space or time. Baker indicated that this pattern could be due to the installation of infrastructure like roads, septic tanks, and concrete culverts that leach a constant source of ions, but might also result from previous stages of construction that would not produce changes in impervious cover.
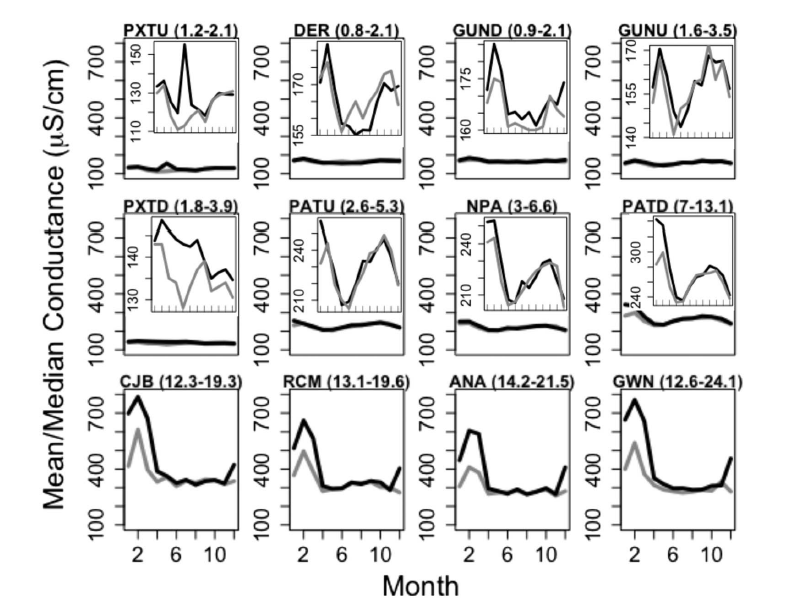
“Monthly (January‐December) median (grey) and mean (black) specific conductance for 12 study watersheds in the Baltimore‐Washington, DC, metropolitan area from 1986 to 2010. The range of impervious cover is shown parenthetically next to each watershed code and panels are organized according to rank 2010 percent imperviousness. Conductivity axes are standardized to facilitate cross‐panel comparison, whereas the first eight panels are inset with unstandardized plots to better illustrate seasonal variation.” (Baker et al 2019).
From 5-13% watershed impervious surface, conductivity became more variable, but oddly enough, did not track additional development or specific watersheds. Instead, variation in conductivity was largely associated with year or season, with wintertime extremes exceeding other times of the year. Baker noted that the lowest concentrations in this range nevertheless remained elevated compared to those at lower levels of development, yet the resulting patterns “appeared more closely related to what people did on impervious surfaces than how much was added”.
At levels of impervious surface above 13%, conductivity remained elevated, yet the degree of variability in conductivity increased dramatically. Because the team observed that conductivity was always higher in winter months and often saw spikes after storm events, they inferred that hydrologic connectivity and the application of road deicers (like road salts) after snowstorms drove the variability.
In more developed watersheds, the authors were surprised to see that conductivity from a winter storm could remain elevated into the following spring or summer, meaning that pulses in the system could take months to be rinsed away. “No matter when the storm happened, conductivity might still be elevated,” explained Baker. “There’s a memory in the system that could last for months.”
Baker found it notable that there were specific thresholds of impervious surface (4-5% and 13%) that altered the relationship between subsequent development and stream chemistry. “We need to understand those signals better,” he said. “They might have implications for future management.”
What does this mean for the Chesapeake Bay?
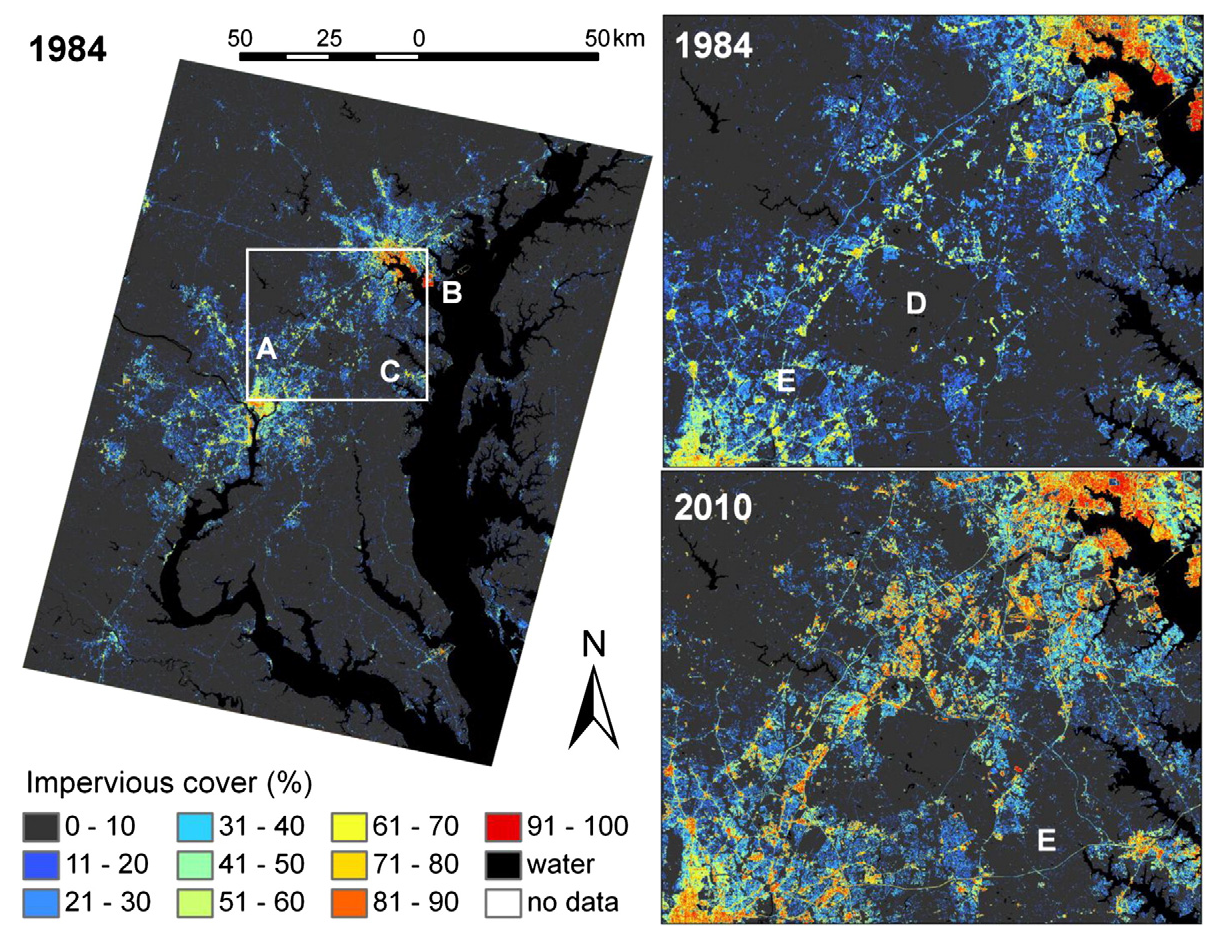
“Impervious surface cover of the study area in 1984, and growth of the D.C.–Baltimore corridor from 1984 to 2010. Points of interest are: (A) Washington, D.C.; (B) Baltimore, Maryland; (C) Annapolis, Maryland; (D) Patuxent Wildlife Research Refuge; and (E) US Highway 50.” Figure from Sexton et al. 2013.
The Bay is already salty, so many of the resident species are tolerant to salinity. Many streams in the Mid-Atlantic, however, are relatively dilute freshwater and Baker warned that even small changes in conductivity could have significant effects on sensitive species like aquatic insects, many of which provide important resources for both freshwater and estuarine food webs.
With that in mind, Baker has thought about how Bay management could tweak its monitoring and restoration efforts to capture and mitigate risks. For example, he explained that a lot of stream restoration focuses on physical or riparian habitat, whereas success is measured by the return of a sensitive species after restoration.
“If salt concentrations are preventing sensitive macroinvertebrates from coming back, none of those physical factors will improve the apparent health of the stream,” Baker explained. In addition to current efforts, Baker recommends that managers take the connections between development, dissolved ions, and invertebrates into account when measuring stream health. This might mean altering when water chemistry is sampled to better capture seasonal patterns. “Most assessment programs measure water chemistry when biotic samples are collected, often in the late spring or early summer. Our work suggests these data may be inadequate for detecting chemical impacts on stream biota.”
Where is the team going from here?
Baker is interested in “developing better measures of biotic responses” to changes in stream conditions. Right now, managers may use metrics measuring biological health of the stream that aren’t telling the whole story. For example, if a manager looks at species richness, or the number of species in a stream, that measure will not capture which species are coming and going, such as losing sensitive species and gaining tolerant species.
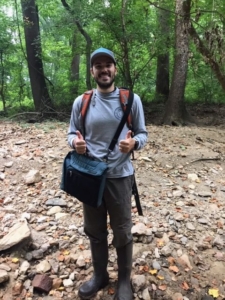
Schley at his current NPS job. Courtesy of Matt Schley.
In 2010, Baker published an approach for measuring the composition of stream communities of invertebrates that was more sensitive than what was typically used by state managers. Now, he and others are building on that work by comparing biological composition to water chemistry. “There’s lots of evidence, both direct and indirect, that chemistry can be a principal driver of assemblage change,” Baker explained.
Schley, who studied under Baker and graduated from UMBC in 2013, is now working for the National Park Service. “This research helped to morph my perspective. Road salts have a direct application in park management during the winter.”
Over the course of his research, Schley noticed that it’s usually assumed that sediment reduction will decidedly help stream chemistry and biology, but “I think that is a bit of a presumptive perspective. This research shows that water chemistry and the biology of the stream may not improve without us actually taking a focused approach to [changing] those parameters.”
On the issue of Bay management, Baker added, “it may be that we’re using freshwater integrity as an indicator of what is likely to protect the Bay, but this salt story [shows a] disconnect, disassociating the integrity of the Bay with that of freshwater ecosystems.” He finished, “the things we need to do to protect the freshwater ecosystems might be different that the things we do to protect the Bay.”